Charging Ahead Strategies for Expanding EV Charging Infrastructure
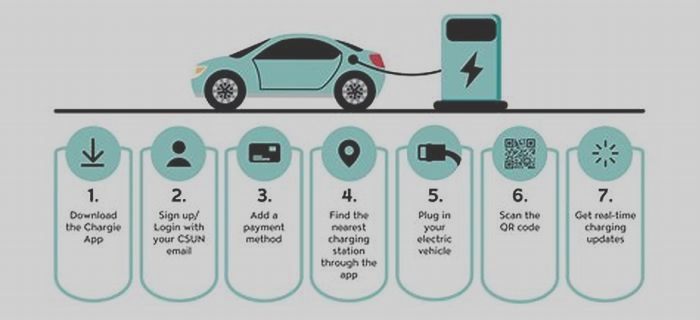
In the regions where electric trucks are becoming commercially available, battery electric trucks can compete on a TCO basis with conventional diesel trucks for a growing range of operations, not only urban and regional, but also in the heavy-duty tractor-trailer regional and long-haul segments. Three parameters that determine the time at which TCO parity is reached are tolls; fuel and operations costs (e.g. the difference between diesel and electricity prices faced by truck operators, and reduced maintenance costs); and CAPEX subsidies to reduce the gap in the upfront vehicle purchase price. Since electric trucks can provide the same operations with lower lifetime costs (including if a discounted rate is applied), the time horizon in which vehicle owners expect to recuperate upfront costs is a key factor in determining whether to purchase an electric or conventional truck.
The economics for electric trucks in long-distance applications can be substantially improved if charging costs can be reduced by maximising off-shift (e.g. night-time or other longer periods of downtime) slow charging, securing bulk purchase contracts with grid operators for mid-shift (e.g.during breaks), fast (up to 350kW), or ultra-fast (>350kW) charging, and exploring smart charging and vehicle-to-grid opportunities for extra income.
Electric trucks and buses will rely on off-shift charging for the majority of their energy. This will be largely achieved at private or semi-private charging depots or at public stations on highways, and often overnight. Depots to service growing demand for heavy-duty electrification will need to be developed, and in many cases may require distribution and transmission grid upgrades. Depending on vehicle range requirements, depot charging will be sufficient to cover most operations in urban bus as well as urban and regional truck operations.
The major constraint to rapid commercial adoption of electric trucks in regional and long-haul operations is the availability of mid-shift fast charging. Although the majority of energy requirements for these operations could come from off-shift charging, fast and ultra-fast charging will be needed to extend range such that operations currently covered by diesel can be performed by battery electric trucks with little to no additional dwell time (i.e. waiting). Regulations that mandate rest periods can also provide a time window for mid-shift charging if fast or ultra-fast charging options are available en route: the EuropeanUnion requires 45minutes of break after every 4.5hours of driving; the UnitedStates mandates 30minutes after 8hours.
Most commercially available direct current (DC) fast charging stations currently enable power levels ranging from 250-350kW. The European Unions Alternative Fuels Infrastructure Regulation (AFIR) aims to enable mid-shift charging across the EUs core TEN-T network, which covers 88% of total long-haul freight activity, and along other key freight corridors. The provisional agreement reached by the European Council and Parliament includes a gradual process of infrastructure deployment for electric heavy-duty vehicles starting in 2025. Recent studies of power requirements for regional and long-haul truck operations in the UnitedStates and Europe find that charging power higher than 350kW, and as high as 1MW, may be required to fully recharge electric trucks during a 30- to 45-minute break.
Recognising the need to scale up fast or ultra-fast charging as a prerequisite for making both regional and, in particular, long-haul operations technically and economically viable, in 2022 Traton, Volvo, and Daimler established an independent joint venture, Milence. With EUR500million in collective investments from the three heavy-duty manufacturing groups, the initiative aims to deploy more than 1700 fast (300 to 350kW) and ultra-fast (1MW) charging points across Europe.
Multiple charging standards are currently in use, and technical specifications for ultra-fast charging are under development. Ensuring maximum possible convergence of charging standards and interoperability for heavy-duty EVs will be needed to avoid the cost, inefficiency, and challenges for vehicle importers and international operators that would be created by manufacturers following divergent paths.
In China, co-developers China Electricity Council and CHAdeMOs ultra ChaoJi are developing a charging standard for heavy-duty electric vehicles for up to several megawatts. In Europe and the UnitedStates, specifications for the CharIN Megawatt Charging System (MCS), with a potential maximum power of 4.5MW, are under development by the International Organization for Standardization (ISO) and other organisations. The final MCS specifications, which will be needed for commercial roll-out, are expected for 2024. After the first megawatt charging site offered by Daimler Trucks and Portland General Electric (PGE) in 2021, at least twelve high-power charging projects are planned or underway in the United States and Europe, including charging of an electric Scania truck in Oslo, Norway, at a speed of over 1MW, Germanys HoLa project, and the Netherlands Living Lab Heavy-Duty and Green Transport Delta Charging Stations, as well as investments and projects in Austria, Sweden, Spain and the United Kingdom.
Commercialisation of chargers with rated power of 1MW will require significant investment, as stations with such high-power needs will incur significant costs in both installation and grid upgrades. Revising public electric utility business models and power sector regulations, co-ordinating planning across stakeholders and smart charging can all help to manage grid impacts. Direct support through pilot projects and financial incentives can also accelerate demonstration and adoption in the early stages. A recent study outlines some key design considerations for developing MCS rated charging stations:
- Planning charging stations at highway depot locations near transmission lines and substations can be an optimal solution for minimising costs and increasing charger utilisation.
- Right-sizing connections with direct connections to transmission lines at an early stage, thereby anticipating the energy needs of a system in which high shares of freight activity have been electrified, rather than upgrading distribution grids on an ad-hoc and short-term basis, will be critical to reduce costs. This will require structured and co-ordinated planning between grid operators and charging infrastructure developers across sectors.
- Since transmission system interconnections and grid upgrades can take 4-8 years, siting and construction of high-priority charging stations will need to begin as soon as possible.
Alternative solutions include installing stationary storage and integrating local renewable capacity, combined with smart charging, which can help reduce both infrastructure costs related to grid connection and electricity procurement costs (e.g.by enabling truck operators to minimise cost by arbitraging price variability throughout the day, taking advantage of vehicle-to-grid opportunities, etc.).
Other options to provide power to electric heavy-duty vehicles (HDVs) are battery swapping and electric road systems. Electric road systems can transfer power to a truck either via inductive coils3 in a road, or through conductive connections between the vehicle and road, or via catenary (overhead) lines. Catenary and other dynamic charging options may hold promise for reducing the uncertainty of system-level costs in the transition to zero-emission regional and long-haul trucks, competing favourably in terms of total capital and operating costs. They can also help to reduce battery capacity needs. Battery demand can be further reduced, and utilisation further improved, if electric road systems are designed to be compatible not only with trucks but also electric cars. However, such approaches would require inductive or in-road designs that come with greater hurdles in terms of technology development and design, and are more capital intensive. At the same time, electric road systems pose significant challenges resembling those of the rail sector, including a greater need for standardisation of paths and vehicles (as illustrated with trams and trolley buses), compatibility across borders for long-haul trips, and appropriate infrastructure ownership models. They provide less flexibility for truck owners in terms of routes and vehicle types, and have high development costs overall, all affecting their competitiveness relative to regular charging stations. Given these challenges, such systems would most effectively be deployed first on heavily used freight corridors, which would entail close co-ordination across various public and private stakeholders. Demonstrations on public roads to date in Germany and Sweden have relied on champions from both private and public entities. Calls for electric road system pilots are also being considered in the China, India, the United Kingdom and the United States.
First national electric vehicle charging infrastructure strategy published
Minister Ryan also announced the new Shared Island Sports Club Scheme, which will open on 30 January, as the first practical roll-out of the strategy. This 15 million scheme from the Shared Island Fund will help sports clubs install electric vehicle charge points in local communities across the island of Ireland, so when people drop off kids or go to the club for their own use, they can charge their car at the same time. This will be just one form of destination charger. Others will be installed in locations like retail centres or tourism spots for example places where people may be driving to.
Charging ahead: Electric-vehicle infrastructure demand
Ask any electric-vehicle (EV) shopper: poor range and limited attractiveness have long been the two biggest bottlenecks to EV uptake.
Now, however, with more than 350 new, feature-laden EV models to debut by 2025, with ranges that increasingly top 200 miles, these attributes pose less of a hurdle. Instead, if consumers purchase EVs at the expected rates in the next five to ten years, a lack of charging infrastructure could become an obstacle to EV adoption.
Recognizing the charging-capacity gap
Consumers rank not having enough access to efficient charging stations as the third most serious barrier to EV purchase, behind price and driving range. Thats according to McKinseys 2016 EV consumer survey of buyers considering battery-powered EV in China, Germany, and the United States. With EV prices declining and ranges expanding, charging could soon become the top barrier.
McKinseys base-case scenario for EV adoption suggests approximately 120 million EVs could be on the road by 2030 in China, the European Union, and the United States (Exhibit 1). The aggressive-case scenario could see that double. Along with different levels of EV adoption across regions, structural considerations will make charging-station demand highly localized. For example, compare a city like Los Angeles, with many single-family low-rise homes that have parking garages, with Manhattan, where high-rise multi-unit apartment dwellings prevail. These two cities will have extremely different EV charging-infrastructure needs.
Big energy demand, but where to charge?
Total charging-energy demand for the EV vehicle population across China, Europe, and the United States could grow dramatically from 2020 to 2030, increasing from roughly 20 billion kilowatt-hours to about 280 billion kilowatt-hours (Exhibit 2). This estimate reflects assumed EV adoption, total miles driven per year, and the average kilowatt-hours required per mile (a miles-per-gallon equivalent). While 280 billion kilowatt-hours sounds like a big number, it represents less than 10 percent of current US energy demand while reflecting the requirements of all four markets.
Unlike traditional, internal-combustion engine (ICE) vehicles, which typically only refuel at gas stations, EVs can recharge at multiple locations in multiple ways. Our model analyzes charging across four use cases that all assume wired plug-in chargers: at home, at work, in public, and on highways for long-distance trips. Other use cases and technologies are emerging. For example, wireless charging or streetlight charging, while potentially viable, are not included in this analysis.
The energy consumed at home and in the workplace will depend on the number of chargers installed and the amount of energy those chargers provide. Home charging will depend on whether EV owners have garages and on their income demographics. Charger penetration at work will predominantly reflect employer choice or regulatory requirements.
However, people do not only use their vehicles to drive to and from work. Approximately 3 to 6 percent of total miles driven involve long-distance trips that average more than 100 miles. Even with a full charge leaving home, most of todays EVs cannot make that round-trip without recharging. This makes the case for long-distance chargers.
Combined home, work, and long-distance charging could in theory cover an EV owners entire energy demand. While potentially true for drivers who use an EV as a second car only for commuting or errands, this scenario is unlikely at scale for several reasons. For instance, drivers without chargers at home or work must charge in public; drivers who exceed their battery range on a given day may need to visit fast-charge stations; and drivers who forget to charge at home or dont have home chargers must rely on other options, making the case for public charging.
From home to work to public charging
People tend to follow a charging hierarchy that starts at home. Most individual passenger cars remain parked for eight to 12 hours at night, and home charging can be easy and often cheaper than charging elsewhere. The reasons: in most countries, residential electricity is cheaper than commercial or industrial electricity, and most charging can happen overnight when off-peak electricity prices are lower.
In a home-centered base case, approximately 75 to 80 percent of EV owners in the United States and European Union should have access to home charging, which should provide up to 75 percent of their energy needs in 2020 (Exhibit 3). The high penetration of single-family homes in states with high EV adoption will drive this demand, particularly in California and the Pacific Northwest. China should have much lower penetration of home charging because there are fewer single-family homes. Even when considering public-centered alternatives, the importance of home charging will remain highly relevant in the United States.
In the European Union, as EVs go mainstream, charging will likely shift toward public options and away from the home over time, with the share of home charging declining from approximately 75 percent in 2020 to about 40 percent by 2030. Thats because more middle- and lower-income households without home-charging options will buy EVs from 2020 onward. In China, public charging will dominate and increase in importance over time, going from 55 to 60 percent in 2020 to approximately 80 percent by 2030. The structural limitations of highly dense urban cities, which have larger proportions of on-street and large-commercial-garage parking, are the catalysts for increased public-charging demand.
In the near term, low levels of public charging should therefore not significantly hinder EV adoption in the European Union and United States. The situation looks different for China, where over half of the energy will come from public sources. Furthermore, the importance of public charging will likely grow stronger by 2030, reinforcing the need for strategies based on target-market needs.
Choosing slow, fast, or superfast charging
The next question beyond where people will charge concerns the type of technology they will use. Three broad categories of EV charging infrastructure exist today:
- Alternate-current (AC) charging, also known as level 1 or level 2. In this system, an in-car inverter converts AC to direct current (DC), which then charges the battery at either level 1 (equivalent to a US household outlet) or level 2 (240 volts). It operates at powers up to roughly 20 kilowatts.
- DC charging, also known as level 3 or direct-current fast charging (DCFC). This charging system converts the AC from the grid to DC before it enters the car and charges the battery without the need for an inverter. Usually called direct-current fast charging or level 3, it operates at powers from 25 kilowatts to more than 350 kilowatts.
- Wireless charging. This system uses electromagnetic waves to charge batteries. There is usually a charging pad connected to a wall socket and a plate attached to the vehicle. Current technologies align with level 2 chargers and can provide power up to 11 kilowatts.
The kilowatt capacity of a charger determines the speed at which the battery receives electricity. AC level 1 and level 2 are most applicable for homes and workplaces because of the long periods cars remain parked and their lower cost: a simple level 2 for a home can cost as little as $500. DCFC chargers are most applicable in situations where time matters, such as on highways and for fast public charging.
Basic AC level 1 and level 2 power will overwhelmingly remain the dominant charging technology through 2030, providing from 60 to 80 percent of the energy consumed. Most of this charging will take place at homes, workplaces, and via slow-charge public stations (Exhibit 4). DCFC will likely play a much larger role in China, which requires more public-charging infrastructure.
Calculating chargings dollars and cents
Based on charging profiles and available technologies, the industry could require approximately 40 million chargers across China, Europe, and the United States, representing an estimated $50 billion of cumulative capital investment through 2030 (Exhibit 5). The US alone will need a cumulative 13 million chargers and approximately $11 billion of investment by 2030. The European Union will need a cumulative 15 million chargers and roughly $17 billion of investment during the same period. In China, the numbers are a cumulative 14 million chargers and $19 billion of investment.
While most chargersover 95 percentwill be in homes and workplaces from a charger-count perspective, the share of capital investment they represent is closer to roughly 70 percent of the total. This reflects the significantly higher cost of faster chargers. On average, a level 2 charger used in a home costs less than $1,000; one used in a workplace or in public can cost between $3,000 and $5,000. A DCFC starts at about $25,000 and, depending on the power capacity, can rise to more than $200,000 for each unit.
Currently, the business cases for home or workplace level 2 chargers are straightforward, given low up-front capital and operating expenses. Making the business case work for public DCFCs is more difficult. The reasons include higher up-front capital, higher operating costs, and currently low utilization. In the near term, this raises a critical question: Who will provide the necessary capital for public charging while utilization rates remain low, particularly in China where the need for public charging is higher?
As electric-vehicle demand looks increasingly likely to grow and EVs emerge as viable alternatives to ICE cars, an ecosystem of industries needs to stack hands on actions that can enable their broader use. Closing the charging gap is one such action, and resolving it will require a concerted, collaborative effort. Thats why finding the answers to the questions raised here should top the agendas of all stakeholders across the EV ecosystem, especially if charging access becomes the number-one impediment to EV penetration. Understanding specific local needs for early demand and adaptation will be the key to making effective targeted investments, matching demand and supply, and enabling quick returns on investments.
This article was originally published in August 2018; some facts and figures were updated in October 2018.