Electric Vehicle Car Sharing A Sustainable Transportation Solution for Cities
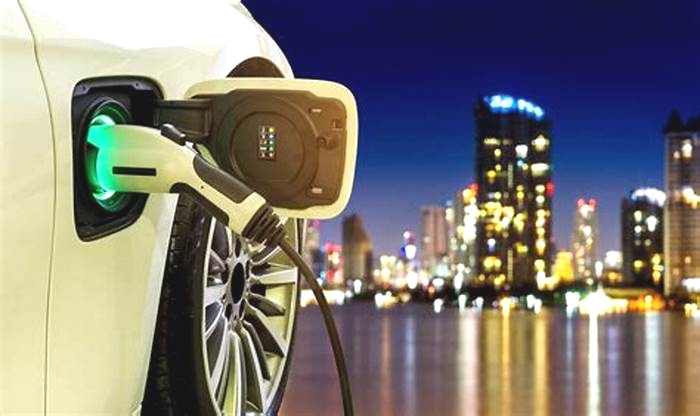
Sustainable operations in electric vehicles sharing: behavioral patterns and carbon emissions with digital technologies
Braun, A., Koch, A., & Hochschild, V. (2016). Intraregionale Unterschiede in der Carsharing-Nachfrage. The Planning Review, 52(1), 7285.
Article Google Scholar
Braun, A., Rid, W. (2018). Assessing driving pattern factors for the specific energy use of electric vehicles: A factor analysis approach from case study data of the Mitsubishi iMiEV minicar. Transportation Research Part D: Transport and Environment, 58, 225238
Chen, T. D., & Kockelman, K. M. (2016). Carsharings life-cycle impacts on energy use and greenhouse gas emissions. Transportation Research Part D: Transport and Environment., 47, 276284.
Article Google Scholar
Chun, Y. Y., Matsumoto, M., Tahara, K., Chinen, K., & Endo, H. (2019). Exploring factors affecting car sharing use intention in the Southeast-Asia Region: A case study in java. Indonesia. Sustainability., 51(03), 11.
Google Scholar
Cruz, C., Palomar, E., Bravo, I., & Aleixandre, M. (2021). Behavioural patterns in aggregated demand response developments for communities targeting renewables. Sustainable Cities and Society, 72, 103001.
Article Google Scholar
Cui, L., Wu, H., Wu, L., et al. (2022). Investigating the relationship between digital technologies, supply chain integration and firm resilience in the context of COVID-19. Annals of Operations Research. https://doi.org/10.1007/s10479-022-04735-y
Article Google Scholar
Deveci, M., Cantez, F., & Gkaar, I. (2018). WASPAS and TOPSIS based interval type-2 fuzzy MCDM method for a selection of a car sharing station. Sustainable Cities and Society, S22106707(17), 3174531746.
Google Scholar
Donateo, T., Licci, F., Delia, A., Colangelo, G., Laforgia, D., & Ciancarelli, F. (2015). Evaluation of emissions of CO2 and air pollutants from electric vehicles in Italian cities. Applied Energy,157, 675687.
Hensher, D. A., Wei, E., & Liu, W. (2021). Battery electric vehicles in cities: Measurement of some impacts on traffic and government revenue recovery. Journal of Transport Geography, 94(3), 103121.
Article Google Scholar
Jaegler, A., & Burlat, P. (2012). Carbon friendly supply chains: A simulation study of different scenarios. Production Planning & Control, 23(04), 269278.
Article Google Scholar
Jia, J., Hao, L., Chen, S., et al. (2022). Vehicles manufacturers pricing strategies considering service level of car-sharing modes. Annals of Operations Research., 315, 17551773.
Article Google Scholar
Jiang, L., Wang, R. Z., Li, J. B., et al. (2018). Performance analysis on a novel sorption air conditioner for electric vehicles. Energy Conversion and Management, 156, 515524.
Article Google Scholar
Jin, F., Yao, E., & An, K. (2020). Analysis of the potential demand for battery electric vehicle sharing: Mode share and spatiotemporal distribution. Journal of Transport Geography., 82, 102630.
Article Google Scholar
Kang, J., Hwang, K., & Park, S. (2016). Finding factors that influence carsharing usage: Case study in Seoul. Sustainability., 8(8), 12.
Article Google Scholar
Kellner, F., & Igl, J. (2012). Estimating the effect of changing retailing structures on the greenhouse gas performance of FMCG distribution on networks. Logistics Research, 4(03), 8799.
Article Google Scholar
Kondor, D., Zhang, H., Tachet, R., Santi, P., & Ratti, C. (2018). Estimating savings in parking demand using shared vehicles for home-work commuting. IEEE Transactions on Intelligent Transportation Systems, 110.
Krishnapuram, B., Carin, L., Figueiredo, M.A.T., & Hartemink, A.J. (2005). Sparse multinomial logistic regression: fast algorithms and generalization bounds. IEEE Transactions on Pattern Analysis and Machine Intelligence, 27, 957968.
Krishna, K., Narasimha M.M. (1999). Genetic K-means algorithm. IEEE Transactions on Systems, Man, and Cybernetics, Part B (Cybernetics), 29, 433439.
Laporte, G., Meunier, F., & Wolfler Calvo, R. (2018). Shared mobility systems: An updated survey. Annals of Operations Research., 271, 105126.
Article Google Scholar
Liao, H., Chen, T. Q., Tang, X., & Wu, J. W. (2019). Fuel choices for cooking in China: Analysis based on multinomial logit model. Journal of Cleaner Production., 225, 104111.
Article Google Scholar
Lin, M., Huang, C., Xu, Z. (2019). MULTIMOORA based MCDM model for site selection of car sharing station under picture fuzzy environment. Sustainable Cities and Society, 101873. https://doi.org/10.1016/j.scs.2019.101873.
Liu, A., Zhao, Y., Meng, X., & Zhang, Y. (2019). A three-phase fuzzy multi-criteria decision model for the charging station location of the sharing electric vehicle. International Journal of Production Economics, 107572.
Liu, N., Lin, J., Guo, S., et al. (2022). Fashion platform operations in the sharing economy with digital technologies: Recent development and real case studies. Annals of Operations Research. https://doi.org/10.1007/s10479-022-04544-3
Article Google Scholar
Luna, T. F., Uriona-Maldonado, M., Silva, M. E., Vaz, C. R. (2020). The influence of e-carsharing schemes on electric vehicle adoption and carbon emissions: An emerging economy study. Transportation Research Part D: Transport and Environment, 79, 102226.
Melliger, M. A., van Vliet, O. P. R., & Liimatainen, H. (2018). Anxiety vs reality Sufficiency of battery electric vehicle range in Switzerland and Finland. Transportation Research Part D: Transport and Environment, 65, 101115.
Article Google Scholar
Meng, J., Mu, Y., Jia, H., Wu, J., Yu, X., & Qu, B. (2016). Dynamic frequency response from electric vehicles considering travelling behavior in the Great Britain power system. Applied Energy, 162, 966979.
Article Google Scholar
Needell, Z. A., McNerney, J., Chang, M. T., & Trancik, J. E. (2016). Potential for widespread electrification of personal vehicle travel in the United States. Nature Energy, 1(9), 16112.
Article Google Scholar
Nijland, H., & van Meerkerk, J. (2017). Mobility and environmental impacts of car sharing in the Netherlands. Environmental Innovation and Societal Transitions., 23, 8491.
Article Google Scholar
Qi, T., & Chen, L. H. (2020). Carsharing: Mitigation strategy for transport-related carbon footprint. Mitigation and Adaptation Strategies for Global Change., 25, 791818.
Article Google Scholar
Ramos, E., Bergstad, C., Chicco, A., Diana, M. (2020). Mobility styles and car sharing use in Europe: attitudes, behaviours, motives and sustainability. European Transport Research Review, 12.
Rangaraju, S., De Vroey, L., Messagie, M., Mertens, J., & Van Mierlo, J. (2015). Impacts of electricity mix, charging profile, and driving behavior on the emissions performance of battery electric vehicles: A Belgian case study. Applied Energy, 148, 496505.
Article Google Scholar
Shen, Y., Zhang, H., & Zhao, J. (2018). Integrating shared autonomous vehicle in public transportation system: A supply-side simulation of the first-mile service in Singapore. Transportation Research Part a: Policy and Practice, 113, 125136.
Google Scholar
Shen, Z. Y., Chen, J. Y., Bai, K. X., Li, Y. X., Cui, Y. X., & Song, M. L. (2023). The digital impact on environmental performance: evidence from Chinese Publishing. Emerging Markets Finance and Trade, 2023, 117.
Article Google Scholar
Song, M. L., Zheng, H. Y., Shen, Z. Y., & Chen, B. Y. (2023a). How financial technology affects energy transformation in China. Technological Forecasting and Social Change, 188, 122259.
Article Google Scholar
Song, M. L., Zhou, W. Z., Upadhyay, A., & Shen, Z. Y. (2023b). Evaluating hospital performance with plant capacity utilization and machine learning. Journal of Business Research, 159, 113687.
Article Google Scholar
Sweet, M. N., & Scott, D. M. (2021). Shared mobility adoption from 2016 to 2018 in the Greater Toronto and Hamilton Area: Demographic or geographic diffusion? Journal of Transport Geography, 96, 103197.
Tayal, A., Gunasekaran, A., Singh, S. P., et al. (2017). Formulating and solving sustainable stochastic dynamic facility layout problem: A key to sustainable operations. Annals of Operations Research, 253, 621655.
Article Google Scholar
Trentini, A., & Losacco, F. (2017). Analyzing carsharing public (scraped) data to study urban traffic patterns. Procedia Environmental Sciences, 37, 594603.
Article Google Scholar
Turo, K., Kubik, A., Chen, F. (2019). Operational aspects of electric vehicles from car-sharing systems. Energies. 12.
Vatanparvar, K., Faezi, S., Burago, I., Levorato, M., & Al Faruque, M. A. (2018). Extended range electric vehicle with driving behavior estimation in energy management. IEEE Transactions on Smart Grid, 10, 29592968.
Article Google Scholar
Wang, G., Makino, K., Harmandayan, A., & Wu, X. (2020). Eco-driving behaviors of electric vehicle users: A survey study. Transportation Research Part D: Transport and Environment., 78, 102188.
Article Google Scholar
Wang, N., & Yan, R. (2016). Research on consumers use willingness and opinions of electric vehicle sharing: An empirical study in Shanghai. Sustainability., 8, 7.
Article Google Scholar
Wappelhorst, S., Dobrzinski, J., Graff, A., Steiner, J., Hinkeldein, D. (2016). Flexible carsharing-potential for the diffusion of electric mobility. Markets and policy measures in the evolution of electric mobility, 6784.
Wielinski, G., Trepanier, M., & Morency, C. (2017). Carsharing versus bikesharing comparing mobility behaviors. Transportation Research Record: Journal of the Transportation Research Board., 2650, 112122.
Article Google Scholar
Xu, Y., & Ji, X. (2018). Study of car-sharing diffusion criticality conditions based on human traveling network. International Journal of Modern Physics C., 29(5), 18400090.
Article Google Scholar
Yang, J., Dong, J., Zhang, Q., Liu, Z., Wang, W. (2018). An investigation of battery electric vehicle driving and charging behaviors using vehicle usage data collected in Shanghai, China. Transportation Research Record: Journal of the Transportation Research Board. 2672.
Yang, J., Wang, W., Ma, K., & Yang, B. (2019). Optimal dispatching strategy for shared battery station of electric vehicle by divisional battery control. IEEE Access 3822438235.
Yoon, T., Cherry, C. R., Ryerson, M. S., & Bell, J. E. (2019). Carsharing demand estimation and fleet simulation with EV adoption. Journal of Cleaner Production., 206, 10511058.
Article Google Scholar
Zhang, B., Chen, H. T., Du, Z .J., Wang, Z. H. (2020). Does license plate rule induce low-carbon choices in residents' daily travels: Motivation and impacts. Renewable and Sustainable Energy Reviews, 124.
Zhang, B., Du, Z. J., Wang, B., & Wang, Z. H. (2019). Motivation and challenges for e-commerce in e-waste recycling under Big data context: A perspective from household willingness in China. Technological Forecasting and Social Change, 144, 436444.
Article Google Scholar
Zhang, B., Niu, N., Li, H., Wang, Z. H., He, W. J. (2021). Could fast battery charging effectively mitigate range anxiety in electric vehicle usage? Evidence from large-scale data on travel and charging in Beijing. Transportation Research Part D: Transport and Environment, 95.
Zhang, B., Xin, Q. Y., Tang, M., Niu, N., Du, H. R., Chang, X. Q., & Wang, Z. H. (2022). Revenue allocation for interfirm collaboration on carbon emission reduction: Complete information in a big data context. Annals of Operations Research, 316, 93116.
Article Google Scholar
Zhang, Y., Chu, L., Fu, Z., Xu, N., Guo, C., Zhang, X., Chen, Z., & Wang, P. (2017). Optimal energy management strategy for parallel plug-in hybrid electric vehicle based on driving behavior analysis and real time traffic information prediction. Mechatronics, 46, 177192.
Article Google Scholar
Zhao, Y., Liu, P., Wang, Z.P., Zhang, L., & Hong, J.C. (2017). Fault and defect diagnosis of battery for electric vehicles based on big data analysis methods. Applied Energy, 207, 354362.
Zou, Y., Yang, C., & Yang, J. (2020). EV-sharing station location and vehicle relocation with parking rewards and punishments mechanism. International Journal of Sustainable Transportation, 15, 306322.
Article Google Scholar
Shared mobility: Sustainable cities, shared destinies
Shared mobility, or when vehicles are shared among individuals over time or together among multiple passengers, appears to be here to stay. Take, for example, the following:
- Consumers racked up more than 15 billion hailed-mobility trips in 2019, with revenues reaching $130 billion. By 2030, total revenues from hailed mobility could increase to between $450 billion and $860 billion, accounting for 80 to 90 percent of consumer spending in shared mobility.
- With a compound annual growth rate (CAGR) of more than 200 percent from 2018 to 2019 in annual yearly trip revenues, the shared-micromobility market is expected to generate up to $90 billion in 2030.
- Fifty-six percent of todays consumers are willing to replace trips taken in private vehicles with future shared autonomous vehicles. As a result of potentially cannibalizing private-vehicle use and providing a more affordable shared-mobility option than e-hailing today, revenue from robo-taxis and robo-shuttles could reach over $400 billion in 2030, depending on how the related regulations and technologies develop.
As consumers demand convenient, cost-effective, and sustainable modes of travel in urban areas, shared mobility is surging. According to a McKinsey analysis of annual reports, the number of e-hailing trips tripled from 5.5 trillion in 2016 to 16.5 trillion in 2019. Over the past decade, shared mobility has also become an attractive field for investors. Since 2010, private investors, technology companies, and others have directed more than $100 billion into shared-mobility companies. Cities are pursuing emissions-cutting goals to address the climate crisis, and this decade may see an even more dramatic shift to flexible, shared, and sustainable ways to travel. More than 150 cities are currently working to introduce measures aiming to reduce private-vehicle use, McKinsey analysis finds.
In a previous article from August 2021, Shared mobility: Where it stands, where its headed, we described seven segments of the shared-mobility market, focusing our analysis on the size of the market, investment trends, and consumer sentiment. We found growing consumer demand for shared mobility, with the number of micromobility trips more than doubling within one year. Our consumer research also revealed that for ride-hailing users, the most important features of shared-mobility services are safety, a competitive price, and availability.
In this article, we offer our perspective on four key segments of shared mobility: hailed mobility, car sharing, shared micromobility, and urban aerial mobility (UAM). We also reveal our projections for sizing up the shared-mobility market in 2030, including estimated global revenues and major trends for each segment. Finally, we present two visions of the future of shared mobility based on varying levels of consumer adoption, regulatory support, and technological advancement.
Shared mobility today and in 2030
To highlight the need for shared mobility, look no further than traditional car ownership. Private-passenger cars are convenient but can also be inefficient. For instance, Germanys passenger-car fleet is around 50 million vehicles, which could potentially provide roughly 250 million seats. With more than 80 million people in Germany, this could theoretically meet the populations mobility needs. But studies show that private vehicles remain parked about 95 percent of the time and often carry a small number of people. (In Europe, an average of 1.2 to 1.9 people occupy passenger cars traveling in urban areas.) This leads to an average utilization of less than 2 percent of all vehicle seat capacity.
Because of this, city streets and highways are often overflowing with traffic, which further reduces effective use of the mobility system.
We segmented the shared-mobility market based on whether rides are pooled with other passengers or strangers, whether consumers are doing their own driving or are being driven, and types of vehicles shared:
Hailed mobility. This segment includes e-hailing (also called ride-hailing), or the individual or pooled use of both licensed and unlicensed driver services, including dynamic shuttle services. In the future, this category will also include shared autonomous vehicles such as robo-taxis and robo-shuttles.
Shared micromobility. A second segment refers to lightweight vehicles such as electric kickscooters, electric bicycles, and electric mopeds (and potentially other options in the future) that are available for shared public use.
Car sharing. A third segment includes car sharing, or when consumers reserve and use company-provided cars, typically for a limited period of time and in one geographic area. Car sharing may be station based, with vehicles returned to a drop-off point, or free floating, meaning that cars can be picked up and returned anywhere. This segment also includes peer-to-peer (P2P) car sharing. This is when car owners charge other drivers to use their vehicles.
Urban aerial mobility. A fourth segment includes flying electric vehicles that transport consumers by air; these can be piloted or flown (semi) autonomously.
Before the COVID-19 pandemic upended public transportation worldwide, global revenues for shared mobility reached roughly $130 billion to $140 billion in 2019 in the United States, Europe, and Greater China, McKinsey analysis shows. At around $120 billion to $130 billion, the ride-hailing segment received the bulk of consumer spending, with the remaining $10 billion mainly split between car sharing and micromobility.
To help cities, automakers, and mobility players understand how the shared-mobility market could evolve, McKinsey developed a Mobility Market Model that includes data from more than 2,800 cities, clustered into 30 different archetypes. Our model projects passenger kilometers traveled in individual cities for more than ten mobility modes, including public transport, private vehicles, and shared mobility. It can also analyze detailed scenarios to help leaders guide the development of shared mobility. Because McKinseys model considers such factors as macroeconomic drivers, cannibalization effects, consumer attitudes, and regulatory effects, it can show how shared mobility may develop within the context of overall mobility.
The shared-mobility market could grow rapidly within the next several years. Depending on customer acceptance of shared mobility, regulations in each country, and the progress of technology, spending on shared-mobility services could reach $500 billion to $1 trillion in 2030, McKinsey analysis finds (Exhibit 1). This would surpass 2019 spending by a magnitude of four to eight and amount to a CAGR of 14 to 19 percent each year from 2019 to 2030.
Ride hailing (including the emergence of shared autonomous vehicles) would likely generate the biggest revenues, as it does today, followed by shared micromobility, car sharing, and UAM. Because UAM is a new segment of shared mobility, its future market size depends on how quickly countries regulate and certify new flying machines, how willing the public is to accept this new way to travel, and how successful organizations are at developing the technology. This could lead to a large variance in future revenue estimates.
Three trends shaping shared mobility
From robo-taxis and robo-shuttles to shared e-bikes, spending on shared-mobility services could grow rapidly over the next decade, with profound implications for policy makers, private companies, and consumers. Three main trends are behind the growth of shared mobility.
The first is a potential transition from individual to pooled use of vehicles. The dizzying pace of urbanization means that congestion is an ongoing challenge in urban areas. Passengers could prefer riding with others because it is cost-effective (with multiple people splitting the bill) and convenient (since ridesharing provides a door-to-door service and consumers do not drive). In addition, cities working to reduce private-vehicle use are enacting stricter regulations and providing incentives for the use of shared mobility. City leaders are establishing car-free zones, charging cars to enter cities, reducing parking spaces, and increasing parking fares. Transitioning to more sustainable, flexible, and pooled modes of travel could provide environmental benefits, reduce traffic, and minimize inefficient use of roads.
The second trend is a possible shift from mobility modes where consumers do their own driving to being drivenfor example, with shared autonomous vehicles. The commercial launch of robo-taxis and robo-shuttles could give consumers more affordable options for point-to-point travel. This might make owning a car unnecessary for some, while for others, reduce how much they use their cars. In turn, value pools could shift away from todays shared-mobility modes into pooled modes along with robo-taxis and robo-shuttles.
A third major trend could involve shifting from using larger to smaller vehicles. Many consumers in crowded areas are having trouble finding parking and no longer want to battle traffic during their daily commutes. Our previous research has shown that almost 70 percent of consumers state that they are willing to use micromobility vehicles for commuting. This suggests that more and more workers are considering using smaller vehicles and more sustainable forms of transportation.
Hailed mobility
The global hailed-mobility segment could reach between $450 billion and $860 billion in 2030, accounting for around 80 to 90 percent of the overall shared-mobility market, based on McKinsey modeling. Within hailed mobility, spending on e-hailing could total as much as $450 billion, while spending on shared autonomous vehicles could reach as much as $410 billion.
The hailed-mobility market is expected to grow 13 to 19 percent each year between 2019 and 2030, with much of this growth propelled by cities that support pooled mobility services. Some European cities have already established low- or zero-emissions zones, and one is also aiming to reduce vehicle kilometers traveled by 27 percent in 2030. In a Norwegian city, officials removed hundreds of parking spots from city streets in 2019. Huge areas are completely car-free, encouraging city dwellers to use public transportation.
The growth of pooled hailed-mobility services is also tied to a consumer mindset shift toward more environmentally friendly modes of travel, and the potential cost advantages of these options. Whats more, new, disruptive hailed-mobility modes could change consumer preferences. The launch of shared autonomous electric vehicles might lead consumers to choose robo-taxis and robo-shuttles over more traditional hailed-mobility modes such as todays e-hailing options, increasing passenger miles traveled and revenue for these vehicles. Depending on the speed of rollout of these vehicles, the transition toward shared mobility and thus the growth of the market could be accelerated even further.
Shared micromobility
Consumer interest in micromobility has flourished during the global COVID-19 pandemic, with many riders valuing hygienic, sustainable, and flexible travel. More than 90 cities have adopted policies that indirectly support micromobility, such as massively building up infrastructure for bicycling, according to McKinsey analysis. Advances in technology will likely further enhance the consumer experiencefor instance, by enabling travel across greater distances. By 2030, the shared-micromobility market could reach $50 billion to $90 billion, a roughly 40 percent increase each year between 2019 and 2030. Shared micromobility could account for around 10 percent of the overall shared-mobility marketin 2030.
Car sharing
The car-sharing market will likely see additional competition by 2030. As robo-taxis and robo-shuttles gain traction, they could provide a more affordable shared-mobility option than driver-based services. The car-sharing market may total between $10 billion to $15 billion in 2030, McKinsey modeling shows. Since consumers who share cars must drive themselves, they have less freedom than they would in an autonomous vehicle. Car-sharing users must find parking, and often have to overcome first- and last-mile gaps (that is, challenges getting from the place where they pick up their car, and then getting from the drop-off point to the final destination). Still, some consumers may appreciate the greater flexibility car sharing offers over car ownership. Mobility operators with purely electric fleets have recently found cities that strictly regulate emissions to be attractive markets.
Urban aerial mobility
UAM is emerging as a new shared-mobility segment. Electric vertical takeoff and landing vehicles (eVTOLs) and short takeoff and landing vehicles (STOLs) might help solve major pain points of on-the-ground travel, including clogged roads and unexpected delays. Rather than spending time in a car stuck in traffic, a business traveler could instead take a short flight to their destination, using the travel time more effectively by catching up on work. According to McKinsey modeling, this market segment could total more than $10 billion in 2030. (More information about this segment and advanced air mobility in general can be found in our Future Air Mobility blog.)
Two visions of shared mobility
The extent to which consumers will increasingly adopt shared mobility, the regulatory support that will materialize, and the technological advances that will occur, particularly for shared autonomous vehicles, remain uncertain. To help stakeholders navigate the emerging landscape, we created two different scenarios that show how the shared-mobility market could evolve over the next decade (Exhibit 2). We focused mainly on cities and urban areas in these scenarios.
Scenario 1: Acceleration of shared mobility, including the use of robo-taxis and robo-shuttles in urban and suburban areas
Our accelerated scenario assumes that in 2030, about two out of five consumers choose to travel sustainably. Cities adopt legislation that strongly drives the growth of pooled ride hailing, and the number of private vehicles on the road drops sharply. Because of this, consumers may likely require more shared-mobility options in a variety of modes. A vertiport (used as a landing pad for UAM aircraft) is available for consumers to transfer seamlessly from one mobility mode to another, including public transit. Private-car ownership declines, roads become much less congested, and far fewer parking lots are needed, freeing up areas for play and recreation. As a result, city dwellers enjoy much more green space, including parks, playgrounds, and public plazas.
In another acceleration, robo-taxis and robo-shuttles are launched and begin to scale, operating in urban and suburban areas. Robo-shuttles undercut private-vehicle prices (and may, therefore, increasingly cannibalize revenue from ride-hailing trips). Consumers highly accept robo-taxis, and cities enact policies to promote the growth of robo-taxi services. In this scenario, the total market potential for shared mobility could reach $1 trillion in 2030.
Scenario 2: Growth continues its current trajectory, and cities become robo-taxi and robo-shuttle hotspots
In our base scenario, which represents the current trajectory, cities enact regulations that modestly drive the growth of pooled ride-hailing. This scenario assumes that one out of five consumers choose sustainable modes of travel, leading to modest gains in public transit and micromobility. Because people are less reliant on private vehicles, fewer parking spots are needed. More space is available for public use, including parks. With fewer cars on the road, not as many lanes are needed, making roads narrower.
Some cities also establish mobility hubs for intermodalityin other words, places where people can easily transfer from one mobility type to anothermaking journeys more seamless. In this scenario, robo-taxis are launched and primarily operate in major cities to transport people and goods. Electric-vehicle chargers, bike lanes, and other infrastructure support sustainable modes of travel. The total market potential for shared mobility could reach $500 billion in 2030 in our base scenario.
Implications for cities, automakers, and mobility players
Cities, automakers, and mobility players may all have to prepare for the mobility transitions that lie ahead, including the potential launch of shared autonomous-car services. Mobility providers may need to adjust to changing market dynamics, and OEMs and suppliers could be required to reinvent vehicles for shared-mobility use. Within cities, officials might consider aligning infrastructure planning to adapt to changing mobility patterns and requirements. Their next steps may involve establishing mobility hubs and allocating space on streets for shared mobility or biking.
Regardless of what scenario materializes, the following steps might help.
Cities. For city officials to achieve their goals of reducing climate-change risk and altering the mix of different transport modes, they will likely need to create policies and provide value propositions that move people from their individual vehicles into shared-mobility offerings at scale. These measures could include proactively promoting micromobility (including shared micromobility), continuing to invest in public transit, and strongly supporting the early adoption of robo-shuttles. As they make these shifts, city planners may want to consider creating more green zones and recreational spaces for public use.
Transportation agencies can look for opportunities to partner with shared-mobility companies and coordinate transit services, potentially even in an integrated offering through mobility-as-a-service. Integrating shared-mobility options such as shared bikes, car sharing, and ride sharing into public-transit systems could allow consumers to plan door-to-door journeys using multiple shared-mobility modes. Offering a multimodal platform could also enable commuters to make more seamless, cost-effective, and environmentally friendly travel plans.
Cities will likely need to define a regulatory framework to establish and promote shared modes of mobility, create appropriate operating boundaries for mobility service providers (MSPs), and set clear targets for passenger miles traveled by mode to ensure that traffic flows smoothly and efficiently. Cities might also consider repurposing existing infrastructure for shared mobility or investing in shared-mobility infrastructure such as lanes for biking and other shared vehicles. Lastly, in collaboration with mobility operators and providers, city officials may want to provide clear guidelines for shared-mobility companies that address, for example, investment in infrastructure and strategies for increasing public use.
Mobility players. MSPs should look for opportunities to collaborate with cities to set goals and build up a mobility ecosystem along the value chain. Mobility players may need to develop customer-centric offerings, including appropriate pricing schemes and a best-in-class technology platform that provides real-time data on services, travel timelines, and costs. Mobility players might also focus on developing integrated shared-mobility offerings that supplement existing services such as public transit. Sharing performance information with city officials may make it easier for shared-mobility companies to fill first- and last-mile gaps. Lastly, MSPs will likely be required to develop clear plans regarding when and how to adopt robo-taxis and robo-shuttles.
The shift in passenger miles traveled from private vehicles to shared-mobility services and public transport is expected to be big. It will likely include massive increases in passenger volumes, requiring MSPs to produce, buy, or lease vehicles that could cover the anticipated rise in demand. To capture significant market share, companies may need to start increasing their fleet sizes today.
Automakers. To capitalize on increasing consumer demand for shared mobility, automotive manufacturers could consider developing vehicles for specific purposes within this market, such as shuttles for pooled ride hailing or shared autonomous vehicles. This may also include three-wheeled vehicles for extended shared-micromobility and microcar offerings. These new concepts might give OEMs the opportunity to capture additional revenue streams in a world of potentially declining car ownership and usage.
Shared mobility has the potential to reshape mobility, benefit the environment, and transform how urban consumers travel as they live, work, and play. How the shared-mobility market develops over the next decade will depend on many factors. But with some planning, cities, automakers, and mobility players can all help usher in a greener, more sustainable future.