Electric Vehicles with the Most Sustainable Materials and Manufacturing Processes
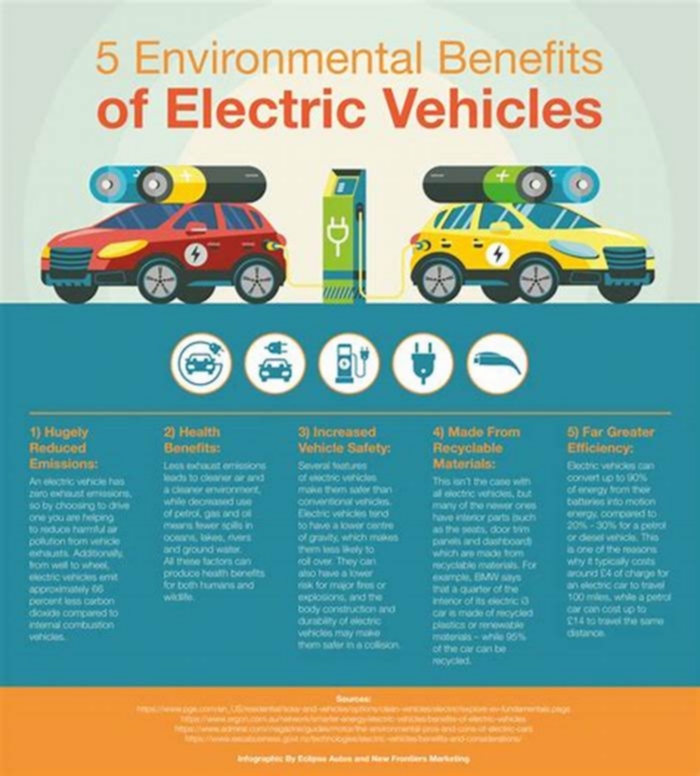
How Green Are Electric Vehicles?
Mining cobalt produces hazardous tailings and slags that can leach into the environment, and studies have found high exposure in nearby communities, especially among children, to cobalt and other metals. Extracting the metals from their ores also requires a process called smelting, which can emit sulfur oxide and other harmful air pollution.
And as much as 70 percent of the worlds cobalt supply is mined in the Democratic Republic of Congo, a substantial proportion in unregulated artisanal mines where workers including many children dig the metal from the earth using only hand tools at great risk to their health and safety, human rights groups warn.
The worlds lithium is either mined in Australia or from salt flats in the Andean regions of Argentina, Bolivia and Chile, operations that use large amounts of groundwater to pump out the brines, drawing down the water available to Indigenous farmers and herders. The water required for producing batteries has meant that manufacturing electric vehicles is about 50 percent more water intensive than traditional internal combustion engines. Deposits of rare earths, concentrated in China, often contain radioactive substances that can emit radioactive water and dust.
Focusing first on cobalt, automakers and other manufacturers have committed to eliminating artisanal cobalt from their supply chains, and have also said they will develop batteries that decrease, or do away with, cobalt altogether. But that technology is still in development, and the prevalence of these mines means these commitments arent realistic, said Mickal Daudin of Pact, a nonprofit organization that works with mining communities in Africa.
Instead, Mr. Daudin said, manufacturers need to work with these mines to lessen their environmental footprint and make sure miners are working in safe conditions. If companies acted responsibly, the rise of electric vehicles would be a great opportunity for countries like Congo, he said. But if they dont, they will put the environment, and many, many miners lives at risk.
The race to decarbonize electric-vehicle batteries
One of the key value propositions of electric vehicles (EVs) for consumersand the planetis their ultralow carbon footprint once in operation. Unlike cars and commercial vehicles with internal-combustion engines, EVs do not produce direct tailpipe emissions from burning diesel and gasoline. But battery-powered EVs have a major emissions challenge of their own: production of the batteries themselves is a highly carbon-intensive process.
Indeed, producing the large lithium-ion batteries used to power EVs is the biggest source of embedded emissions for both electric cars and trucks, accounting for about 40 to 60 percent of total production emissions, according to our estimation. In other words, making batteries can generate as much emissions as producing all the other materials that go into making an EVor even more (Exhibit 1).
As pressure to decarbonize increases and as demand for EVs picks up globally, manufacturers are racing to address this emissions challenge. More than 100 auto industry OEMs and their suppliers have committed to reducing emissions as part of the Science Based Targets initiative. Additional industry leaders are also expected to join this group.
Individual OEM decisions can make a substantial difference. Emission levels from EV battery production depend on a variety of factors, including design choices, vehicle type, range, and freight requirements, as well as production and sourcing locations. The energy sources used to produce various battery components are one of the biggest factors explaining the wide variation in the carbon footprint of different OEMs.
The good news is that steep reductions in the carbon emissions from EV battery production are possible in the next five to ten years. This article looks at why EV battery production is such a high-emissions activity and what can be done to shrink its carbon footprint.
Why EV batteries have such a large carbon footprint
An EV has roughly double the production footprint of a typical internal-combustion-engine (ICE) vehicle. Both have similar embedded production emissions from, for example, producing the body of the vehicle, which is between five and ten tons of CO2e emissions, depending on its size and production location. On top of that, however, producing a typical EV (with a 75-kWh battery pack) emits more than seven tons of CO2e emissions on the battery alone.
The materials and energy needed to produce EV batteries explain much of its heavy carbon footprint. EV batteries contain nickel, manganese, cobalt, lithium, and graphite, which emit substantial amounts of greenhouse gases (GHGs) in their mining and refining processes. In addition, the production of anode and cathode active materials requires high, energy-intensive temperatures for some processes. Battery chemistry, production technology, the selection of raw-material suppliers, and transportation routes are other determining factors for the amount of embedded production carbon.
Sourcing decisionsincluding for the energy usedhave a large impact on emissions, depending on whether renewable energies such as solar and wind power or fossil fuels such as natural gas are used. Producers using renewable electricity already have a significantly smaller carbon footprint in their battery production than those using fossil fuels. Exhibit 2 highlights the wide variation in battery-related emissions depending on value chain choices.
For now, most batteries are manufactured in Asia: China dominates the market with a market share of more than 70 percent and has the most emission-intensive production processes. By contrast, Sweden has maintained a relatively low level of emissions from battery production, averaging less than half that of China.
More battery producers have established capacity in Europe, which has helped drive down the global average for emissions per kWh because electricity has a lower carbon intensity in Europe than in most Asian countries, due to a higher share of renewable-energy sources. Assuming the global push toward decarbonizing electricity grids continues, including in China, our model suggests that the global average of GHGs from battery production could decline to 85 kg CO2e/kWh by 2025. This reduction would come about largely as a result of less emission-intense power generation in the grids of the battery-producing countries.
A growing number of OEMs expect that low-carbon battery production will become a competitive advantage. Some leading players already aim to cut emissions below 20 kg CO2e/kWhor up to almost ten times less than the most emission-intensive OEMs today. Any continuing spread between the best- and worst-in-class performers will provide opportunities for leaders to differentiate their offerings.
To take the lead in low-carbon products, manufacturers of battery pack cells and active materials will need to consider not only decarbonizing their own operations but also addressing the emissions from the materials and components they purchase from their suppliers.
Significant emission reductions by 2030 are feasible
According to our estimates, producing the average EV battery today emits up to 100 kilograms (kg) of CO2 equivalent per kilowatt-hour (CO2e/kWh).
Ambitious players have the ability to reduce the carbon footprint of battery production by up to 75 percent on average in the next five to seven years, but doing so will require action across the entire value chain.
Various strategies can help with abatement. Its costs will depend heavily on existing technologies and external factors such as geography. Some of these strategies will save costs, while others will come at a considerable premium. Key factors are influencing how competitive low-carbon batteries can include production location and target market. In some advantageous cases, it might be possible to decarbonize up to 80 percent at a minimum additional cost to the end customer.
Regulatory shifts such as the European Unions Carbon Border Adjustment Mechanism (CBAM) and the Inflation Reduction Act (IRA) in the United States can focus attention on the changes needed and potentially help reduce the technology costs to achieve them. The CBAM, for instance, is a border tax that makes importing high-carbon products into the European Union more expensive. This may give local low-carbon players a competitive advantage even if they have a higher cost basis for production. In the United States, the IRA subsidizes the local production of batteries as well as the components needed to make them. Part of the subsidy is granted if producers comply with local content requirements, so a certain percentage of minerals can come only from the United States or countries that the United States has a free-trade agreement with. This requirement directly encourages more local production or recycling of minerals and components and indirectly leads to more sustainable batteries.
Regulations are also increasingly providing incentives for OEMs to decrease battery emissions. For instance, the recently agreed EU sustainable-battery strategy will introduce carbon footprint labeling by 2024 and mandate other sustainability requirements such as recycled content, performance, and durability. Consequently, battery manufacturers could see increasing pressure from customers to reduce the emissions embedded in the battery supply chain.
The largest impact across any of these strategies would come from switching to renewable electricity sources or initiating green power purchase agreements (PPAs) in every link of the value chain. For full emissions reduction from electricity, the type and quality of the PPA are important.
Reducing emissions with technology
Specific technology alternatives can reduce emissions and, in some cases, save costs (Exhibit 3). Areas with the most impact include the following:
Raw-material extraction and refining. On average, mining and refining raw materials accounts for about a quarter of total battery production emissions, with lithium and nickel responsible for more than half of that. Emissions of battery-grade nickel vary by a factor of about ten. Location, ore type, and processing technology explain this wide variation. Procuring the metal from sustainable producersthose that might already have switched to electrified mining equipment or renewable-energy sources for electricity, for examplecan result in an emissions reduction in some cases of up to 30 percent per battery cell created.
Active-material (anode and cathode) manufacturing. For both cathode active materials and anode active materials, most emissions come from high-temperature processing. In these steps, boilers and electricity are used to precipitate and dry materials and expose them to strong heat for several hours. Because these processes require electricity, extra effort is needed to ensure process stability and continuity. A quick win would involve switching current electricity consumption to an around-the-clock clean PPA with 100 percent matching of supply and demand; this would reduce as much as 25 percent of total mine-to-cell manufacturing emissions.
Cell manufacturing. Companies can completely electrify the production process. Most nonelectricity emissions in cell manufacturing today come from the electrode-drying process, which requires medium-temperature heat between 50C and 160C. Typical cell manufacturers use natural gasfired electrode drying lines, but electrified versions of this technology exist. Additionally, innovations such as dry coating or switching from conventional binders such as polyvinylidene fluoride (PVDF), a specialty plastic, to water-soluble alternatives during electrode manufacturing could significantly curb energy consumption and related emissions and cost. Supplying a completely electrified cell manufacturing process with 24/7 low-carbon electricity results in, on average, a 25 percent reduction of total mine-to-cell manufacturing emissions.
Using production-adjacent factors to reduce emissions
Additional measures beyond addressing the primary production process can also make a difference. These include using recycled materials rather than virgin raw materials, improving the logistics emissions along the supply chain, choosing the chemistry of the materials used for the battery, and potentially even rethinking the size of batteries themselves.
Recycling. Recycling is not only a long-term remedy for the likely future shortage of raw battery materials such as lithium and nickel but also a fundamental lever to decrease battery emissions and reduce the dependency of EU and US markets on carbon-intensive mining regions. With many new battery factories ramping up globally, large volumes of production scrap will become available, increasing the relevance of a functioning recycling value chain even before larger numbers of EVs reach their end of life in five to ten years. Today, the carbon footprint of recycled battery materials is typically four times smaller than that of raw materials from primary sources. Increasing the share of recycled materials in production is thus an important step toward decarbonization.
Logistics. Typically, only a small portion of battery GHG emissionsabout 5 percent of the overall footprintoriginates from transport of battery cells or their components. The ongoing decarbonization of the transport sector and a switch to low-emission transport modes such as trains will be needed for deep decarbonization. In addition, increased momentum in the localization of the battery value chain could drive down emissions in automotive production regions such as the European Union and the United States.
Chemistry. Today, cell manufacturers and OEMs choose between high-performing nickel-manganese-cobalt (NMC) and lithium-iron-phosphate (LFP) cells. Our analysis suggests that while NMC batteries have a 30 to 40 percent higher energy density, LFP cells have a larger expected charging-cycle lifetime and, on average, 15 to 25 percent lower carbon emissions. This is primarily driven by fewer embedded material emissions in the cathode. Several OEMs, cell producers, and cathode manufacturers are looking into alternative chemistries to reduce emissions and costs while maintaining or increasing energy density. When producing lithium-nickel-manganese-oxide cathodes (LNMO), for example, the goal is to substitute expensive and emission-intensive materials such as nickel with cheaper, abundant, and more sustainable materials such as manganese.
Battery size. For now, EV producers are focusing on increasing battery pack sizes to enable drivers to travel longer distances. In 2021, the EV with the longest range reached 405 miles (652 kilometers [km]) on a single battery charge. In 2022, the number of EVs with a range farther than 300 miles (483 km) tripled in the United States. Yet there is a mismatch between growing battery sizes and the distances that average drivers travel daily, which is less than 40 miles (64 km) in the United States. According to the Federal Highway Administration (FHWA), 95 percent of all trips were less than 30 miles (48 km)less than one-tenth the distance the longest-range EV can travel on a single charge. Because of this disconnect between innovation and application, the limited resources allocated to battery production are largely underused. One radical way to reduce emissions would therefore be to build smaller battery packs tailored more toward consumer needs. In China, for instance, the best-selling electric vehicle in 2021 was the Wuling Hongguang Mini EV, which has a nine to 14 kWh battery offering and a range of 75 to 106 miles (121 km to 171 km).
Successful decarbonization requires strategic collaboration along the value chain
To build zero-carbon batteries, players along the entire value chain need to work together and with other stakeholders, including governments and financiers. To succeed, they should consider action in five areas:
Suppliers. Producers can set a clear demand signal for zero-carbon products along the value chain to suppliers. For example, a best-in-class EV OEM could send a signal to its cell suppliers, which in turn can relay that demand to its active-material suppliers, and so on, until the demand for such materials finds its way up the value chain to raw-material mining and refining. This can be done by establishing procurement partnerships to jointly develop low-carbon solutions or increase demand for low-carbon products.
Investors. Stakeholders can consider helping innovators with their investments by securing financing. For example, they might use long-term volume commitments for sustainable production to build new low-emission production technology. Public subsidies could potentially be beneficial in achieving these goals, if governments are willing to consider them.
Recycling. Players across the value chain could scale up battery collection and recycling, including logistics, testing and disassembly, processing, and digital track and trace. As described above, increasing the share of recycled materials in new battery cells would not only help address the expected supply shortage for battery materials but also significantly reduce the CO2e footprint of such batteries.
Metrics. Producers could increase transparency by establishing standards and metrics. One option would be a battery passport, which was recently launched by the Global Battery Alliance. Other options include second-life standards, or certifications for low-carbon products that make it easier for customers to choose low-carbon options and track improvements along the value chain.
Partnerships. Players along the value chain may want to form multilateral partnerships. For instance, a partnership between raw-material companies (such as nickel, cobalt, lithium, and aluminum), active-material producers, cell manufacturers, and OEMs could help address issues along the entire value chain. Such partnerships might consider making a joint commitment to switch to renewable electricity sources in each step of the value chain, for example.
How companies could get started today
EV battery companies seeking to start decarbonizing their own upstream emissions would need to create a playbook. A first step could be to create a comprehensive overview of the carbon footprint of their own product based on a detailed understanding of their upstream emissions. This overview could showcase their supplier portfolio and other players they work with along the value chain. Companies could accomplish this by collecting primary data from their suppliers (and in turn their suppliers suppliers) and assessing the decarbonization options available today and over time. This information and transparency would help set the right ambition level and help companies choose the right strategy based on careful consideration of differentiation opportunity, cost, and risk.
As a possible follow-up, companies may want to build a concrete action plan to reach their goals, including quick wins such as switching to renewable-energy sources and long-term strategic actions across their supply chain. Strategic actions could include establishing alliances and partnerships with relevant players along the value chain. Finally, companies could develop a strategy for positioning themselves in front of CO2-aware customers and look for ways to differentiate themselves from competitors, potentially extracting sustainability price premiums in the medium term.
In the race to reduce emissions generated by EV battery production, OEMs have many options for getting ahead. The technologies are either in place or rapidly emerging and will enable them to substantially reduce the carbon footprint of batteries. Doing so will ensure that electric vehicles live up to the hopes that many consumers place in them and mark a breakthrough in the larger race to decarbonize mobility and the economy as a whole.