Solving EV Range Anxiety Strategies for Long Distance Travel
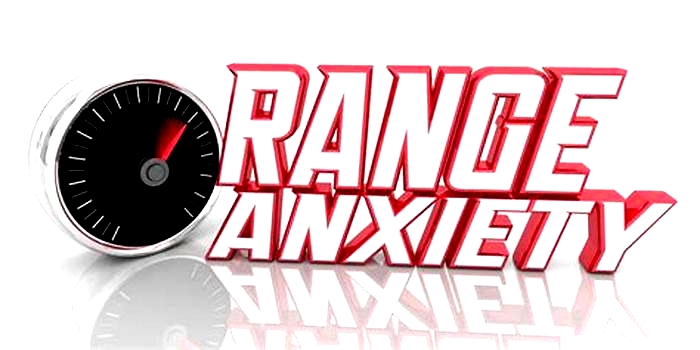
Addressing the range anxiety of battery electric vehicles with charging en route
Sources of Greenhouse Gas Emissions. https://www.epa.gov/ghgemissions/sources-greenhouse-gas-emissions. (U.S. Environmental Protection Agency, Accessed November 12, 2021).
Emissions from Hybrid and Plug-In Electric Vehicles. https://afdc.energy.gov/vehicles/electric_emissions.html. (Alternative Fuels Data Center, Accessed November 12, 2021).
Andwari, A. M., Pesiridis, A., Rajoo, S., Martinez-Botas, R. & Esfahanian, V. A review of battery electric vehicle technology and readiness levels. Renew. Sustain. Energy Rev. 78, 414430 (2017).
Article Google Scholar
Hoffart, F. Proper care extends Li-ion battery life. Power Electron. 25 (2008).
Costs Associated With Non-residential Electric Vehicle Supply Equipment. https://afdc.energy.gov/files/u/publication/evse_cost_report_2015.pdf. (U.S. Department of Energy, 2015).
Frances Solar Road Dream May be Over After Test Fails. http://www.globalconstructionreview.com/news/frances-solar-road-dream-may-be-over-after-test-fa/. (Global Construction Review, 2019).
Bulut, E., Kisacikoglu, M. C. & Akkaya, K. Spatio-temporal non-intrusive direct v2v charge sharing coordination. IEEE Trans. Veh. Technol. 68, 93859398 (2019).
Article Google Scholar
You, P. & Yang, Z. Efficient optimal scheduling of charging station with multiple electric vehicles via v2v. In 2014 IEEE International Conference on Smart Grid Communications (SmartGridComm), 716721 (IEEE, 2014).
Kosmanos, D. et al. Route optimization of electric vehicles based on dynamic wireless charging. IEEE Access 6, 4255142565 (2018).
Article Google Scholar
Pham, T. S. et al. Optimal frequency for magnetic resonant wireless power transfer in conducting medium. Sci. Rep. 11, 111 (2021).
Article Google Scholar
Chakraborty, P., Parker, R., Hoque, T., Cruz, J. & Bhunia, S. P2c2: Peer-to-peer car charging. In 2020 IEEE 91st Vehicular Technology Conference (VTC2020-Spring), 15 (IEEE, 2020).
Krajzewicz, D., Erdmann, J., Behrisch, M. & Bieker, L. Recent development and applications of SUMO-Simulation of Urban MObility. Int. J. Adv. Syst. Meas. 5, 3&4 (2012).
Alvaro-Hermana, R., Fraile-Ardanuy, J., Zufiria, P. J., Knapen, L. & Janssens, D. Peer to peer energy trading with electric vehicles. IEEE Intell. Transp. Syst. Mag. 8, 3344 (2016).
Article Google Scholar
Wang, M. et al. Spatio-temporal coordinated v2v energy swapping strategy for mobile pevs. IEEE Trans. Smart Grid 9, 15661579 (2016).
Article Google Scholar
Zhang, R., Cheng, X. & Yang, L. Flexible energy management protocol for cooperative ev-to-ev charging. IEEE Trans. Intell. Transp. Syst. 20, 172184 (2018).
Article Google Scholar
Shurrab, M., Singh, S., Otrok, H., Mizouni, R., Khadkikar, V., & Zeineldin, H., A stable matching game for v2v energy sharing-a user satisfaction framework. IEEE Trans. Intell. Transp. Syst. (2021).
Kabir, M. E., Sorkhoh, I., Moussa, B. & Assi, C. Joint routing and scheduling of mobile charging infrastructure for v2v energy transfer. IEEE Trans. Intell. Veh. 6(4), 736746 (2021).
Kong, P.-Y. Autonomous robot-like mobile chargers for electric vehicles at public parking facilities. IEEE Trans. Smart Grid 10, 59525963 (2019).
Article Google Scholar
Zhang, M. et al. A unified configurational optimization framework for battery swapping and charging stations considering electric vehicle uncertainty. Energy 218, 119536 (2021).
Article Google Scholar
Wu, H., Pang, G. K. H., Choy, K. L. & Lam, H. Y. An optimization model for electric vehicle battery charging at a battery swapping station. IEEE Trans. Veh. Technol. 67, 881895 (2017).
Article Google Scholar
Lu, L., Han, X., Li, J., Hua, J. & Ouyang, M. A review on the key issues for lithium-ion battery management in electric vehicles. J. Power Sources 226, 272288 (2013).
Article ADS CAS Google Scholar
Koufakis, A.-M., Rigas, E. S., Bassiliades, N. & Ramchurn, S.D. Towards an optimal ev charging scheduling scheme with v2g and v2v energy transfer. In 2016 IEEE International Conference on Smart Grid Communications (SmartGridComm), 302307 (IEEE, 2016).
Etezadi-Amoli, M., Choma, K. & Stefani, J. Rapid-charge electric-vehicle stations. IEEE Trans. Power Deliv. 25, 18831887 (2010).
Article Google Scholar
Afshar, S., Macedo, P., Mohamed, F. & Disfani, V. Mobile charging stations for electric vehiclesA review. Renew. Sustain. Energy Rev. 152, 111654 (2021).
Article Google Scholar
Zhang, Y. et al. Mobile charging: A novel charging system for electric vehicles in urban areas. Appl. Energy 278, 115648 (2020).
Article Google Scholar
Conway, P., Kalyanasundharam, N., Donley, G., Lepak, K. & Hughes, B. Cache hierarchy and memory subsystem of the AMD opteron processor. IEEE Micro 30, 1629 (2010).
Article Google Scholar
Rahman, R. A., Tamin, M. N. & Kurdi, O. Stress analysis of heavy duty truck chassis as a preliminary data for its fatigue life prediction using fem. J. Mek. 26 (2008).
ORCA Inceptive data sheet. http://andromedapower.com/uploads/ORCA_Inceptive_Datasheet.pdf. (Andromeda Power LLC., 2019).
Mobile Connector Owners Manual. https://www.tesla.com/sites/default/files/downloads/gen_2_mobile_connector_owners_manual_32_amp_en_US.pdf. (Tesla, 2012).
Tesla impact report 2019. https://www.tesla.com/ns_videos/2019-tesla-impact-report.pdf. (Accessed March 26, 2021).
Greenhouse Gases Equivalencies Calculator|US EPA. https://www.epa.gov/energy/greenhouse-gases-equivalencies-calculator-calculations-and-references. (Accessed March 26, 2021).
Stages, L. C. et al. Life cycle greenhouse gas emissions from solar photovoltaics. J. Ind. Ecol. (2012).
Sullivan, J., Burnham, A., Wang, M. etal. Energy-consumption and carbon-emission analysis of vehicle and component manufacturing. (Technical Report, Argonne National Laboratory (ANL), 2010).
Automotive Trends Report|US EPA. https://www.epa.gov/automotive-trends/highlights-automotive-trends-report. (Accessed March 26, 2021).
Bates, J. & Leibling, D. Spaced Out: Perspectives on Parking Policy. (2012).
Shoup, D. The High Cost of Free Parking: Updated Edition (Routledge, 2017).
Moeini, A. & Wang, S. Design of fast charging technique for electrical vehicle charging stations with grid-tied cascaded h-bridge multilevel converters. In 2018 IEEE Applied Power Electronics Conference and Exposition (APEC), 35833590 (IEEE, 2018).
Bergenhem, C., Shladover, S., Coelingh, E., Englund, C. & Tsugawa, S. Overview of platooning systems. In Proceedings of the 19th ITS World Congress (2012).
Swaroop, D. & Hedrick, J.K. Constant spacing strategies for platooning in automated highway systems (1999).
Tsugawa, S., Jeschke, S. & Shladover, S. E. A review of truck platooning projects for energy savings. IEEE Trans. Intell. Veh. 1, 6877 (2016).
Article Google Scholar
Dey, K. C. et al. A review of communication, driver characteristics, and controls aspects of cooperative adaptive cruise control (CACC). IEEE Trans. Intell. Transp. Syst. 17, 491509 (2015).
Article Google Scholar
Wang, Z., Wu, G. & Barth, M.J. A review on cooperative adaptive cruise control (CACC) systems: Architectures, controls, and applications. In 2018 21st International Conference on Intelligent Transportation Systems (ITSC), 28842891 (IEEE, 2018).
Gong, S. & Du, L. Cooperative platoon control for a mixed traffic flow including human drive vehicles and connected and autonomous vehicles. Transp. Res. Part B Methodol. 116, 2561 (2018).
Article Google Scholar
Gong, S., Shen, J. & Du, L. Constrained optimization and distributed computation based car following control of a connected and autonomous vehicle platoon. Transp. Res. Part B Methodol. 94, 314334 (2016).
Article Google Scholar
Liang, K.-Y., Mrtensson, J. & Johansson, K. H. Heavy-duty vehicle platoon formation for fuel efficiency. IEEE Trans. Intell. Transp. Syst. 17, 10511061 (2015).
Article Google Scholar
Ma, H., Chu, L., Guo, J., Wang, J. & Guo, C. Cooperative adaptive cruise control strategy optimization for electric vehicles based on SA-PSO with model predictive control. IEEE Access 8, 225745225756 (2020).
Article Google Scholar
Zhang, H., Du, L. & Shen, J. Hybrid MPC system for platoon based cooperative lane change control using machine learning aided distributed optimization. Transp. Res. Part B Methodol.https://doi.org/10.1016/j.trb.2021.10.006 (2021).
Article Google Scholar
Shen, J., Kammara, E. K.H. & Du, L. Fully distributed optimization-based CAV platooning control under linear vehicle dynamics. Transp. Sci. (2022).
Luu, D.L., Lupu, C., Ismail, L.S. & Alshareefi, H. Spacing control of cooperative adaptive cruise control vehicle platoon. In 2020 IEEE International Conference on Automation, Quality and Testing, Robotics (AQTR), 16 (IEEE, 2020).
Rajamani, R., Tan, H.-S., Law, B. K. & Zhang, W.-B. Demonstration of integrated longitudinal and lateral control for the operation of automated vehicles in platoons. IEEE Trans. Control Syst. Technol. 8, 695708 (2000).
Article Google Scholar
Guanetti, J., Kim, Y. & Borrelli, F. Control of connected and automated vehicles: State of the art and future challenges. Annu. Rev. Control 45, 1840 (2018).
Article MathSciNet Google Scholar
How to say goodbye to EV range anxiety
Robin van den Berg argues that real-time information such as live maps and traffic updates can help address range anxiety
A cleaner, more sustainable future is in sight as interest in electric vehicles (EVs) continues to soar. But a vital question still remains: how can we make adopting EVs safe and easy for everyone?
With Norwayholding the largest percentage of EVs in Europe and the Netherlandsholding the highest density of public charging stations across Europe, more needs to be done to ensure other countries follow suit. Despite plug-in sales growing by 43% against a global light vehicle decline of 14%, this growth is still largely concentrated in specific regions andelectrics total share of the light vehicle market is still only about 4%.
The environmental benefits behind EV uptake cant be challenged.Road transport pollution is responsible for over 20%of all CO2 emissions caused by humans. EVs reduce harmful vehicle emissions by up to 30% when operating with electricity generated from fossil fuels, and this figure grows beyond 70% when using renewable energy sources. With so many reasons behind going electric, why are consumers still hesitant to make the move? It mostly comes down to one sticking point: EV range anxiety.
Range anxiety explained
EV range anxiety is the fear of running out of power on a journey and not being able to find a charging point. Its a major stumbling block to greater EV adoption, and it has more to do with psychology than the actual range of EVs or the availability of charging points. The range of EVs has increased by roughly 15% on average in the last few years and the infrastructure for physical charging points is constantly expanding.
EV range anxietyhas more to do with psychology than the actual range of EVs or the availability of charging points
Range anxiety helps explain why, despite the regulatory push from governments and technology improvements from manufacturers, most vehicles are still sold with a combustion engine. Were still waiting to see the mass groundswell of adoption that the industry needs.
Plugging in and charging up
Despite economic uncertainty, EV sales continue to rise. This is encouraging, as the benefits of a sustainable and cleaner way of moving will be shared by everyone, not only by early adopters or even just EV drivers, but for all citizens of the world. This is one of the reasons why governments are incentivising EV adoption, such asUS president Bidens proposal for US$174bn in EV incentives, and theUKs proposed ban on new diesel and gasoline cars from 2030. Similarly, automakers are announcing the phasing out of combustion-engine vehicles by 2035, 2030 or even as early as 2025.
However, to make sure this transition is a success, the experience must be great for all users, not just early adopters. This is particularly important for existing vehicle manufacturers, asa study from JD Powersuggests that dissatisfied vehicle owners will be nearly three times as likely to not repurchase the same brand. The same study also indicates that, to ensure a good experience, the driver needs reliable range prediction, plus good support for charging stations.
Finding your range
Just how far can an EV go on a single charge?The average EV range is about 200 miles or 320 km, which means most day-to-day drives are well within an EVs range, whether its a short commute, trip to the shops or seeing friends and relatives. For most people, journeys beyond an EVs range are only made once or twice a yearon annual holidays or seasonal trips around the new year.
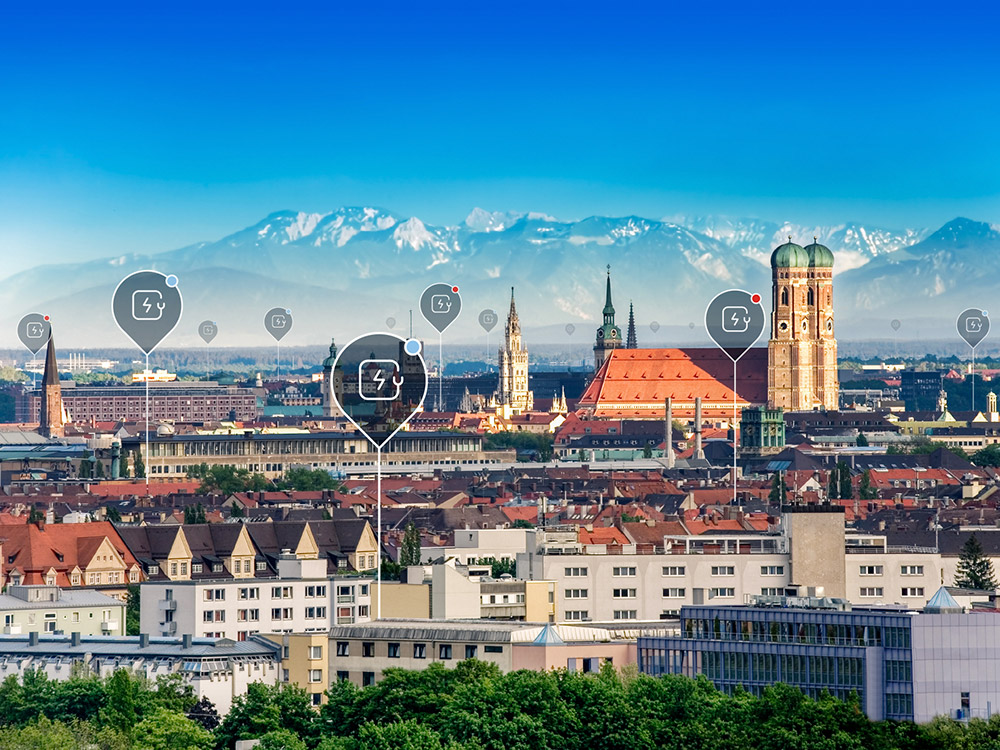
But widespread charging station networks are still essential, not only because of the expected growth in EV usage, but also because of the psychological value. Ubiquitous access helps drive EV adoption. The Netherlands, one of the best places in Europe to drive an EV, has recognised this. Its a relatively small country with many destinations nearby, yet the government is stimulating the infrastructure network and installing numerous EV charging points. It also helps EV owners directly by installing charging points close to an EV owners home, upon request, if theres not one already nearby.
Elevation, weather, traffic and, most of all, driving speed play a role in an EVs achievable range on a single charge. When planning a route, its vital to know the driving conditions on the day and the style of the driver behind the wheel. Having a heavy accelerator foot will have a large effect on an EVs effective range.
Using this data, technology can help provide accurate range calculations for more predictable (and less anxiety-inducing) journeys. Knowing the information in advance is vital to strategic and safe EV route planning: being able to skip a charging stop could mean shaving 30 minutes off your ETA. Providing accurate range information is therefore essential to increase trust in EV adoption.
Going the extra mile
EV drivers need peace of mind. That involves taking the psychological obstacle of range anxiety away from EV driving. This can be achieved by drivers understanding the best way to reach any destination, within or beyond a vehicles range. Long-distance EV routing will help show drivers where and when to charge on long journeys, as well as how long the stop will take.
Having in-dash navigation systems with connected services technology will provide drivers with the best user experience. It tells drivers if their favourite destinations can be reached without having to recharge and shows the charging options available to them based on compatibility with their vehicles and real-time availability of charging points.
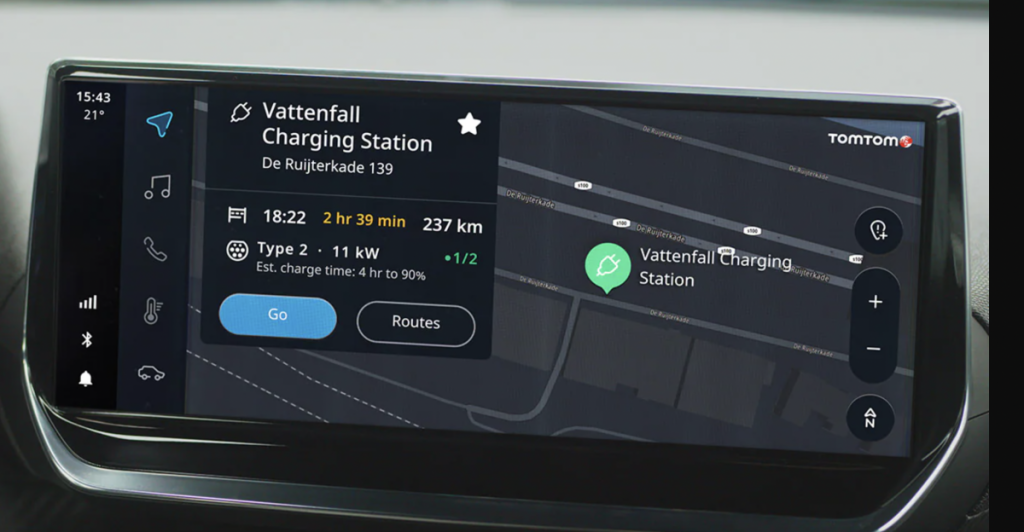
Advanced road knowledge is vital for car manufacturers. With a clear view of the road ahead, an EV can anticipate and optimise its energy management. For instance, if the driver has planned a route to a fast-charge station through the in-vehicle navigation system, the EV can use this knowledge to start pre-conditioning the battery to achieve the optimal temperature for charging upon arrivalimproving charging speed. A hybrid vehicle can also draw more power from the battery going uphill, if it knows it can recuperate charge going downhill on the other side.
Mapping the road ahead
Drivers feel confident when they can anticipate the road ahead. Providing them with clear route planning, visibility of charging stations and available charging points will reassure drivers about their upcoming journeys, removing much of the doubt and psychological barriers around driving an EV.
Having real-time information to hand, such as live maps and traffic updates that identify any upcoming bumps, curves and jams in the road will also add to the reassurance that the car can handle the range. Rest assured; the EV revolution is well under way. We just need to ensure both the technology and infrastructure are ready for all types of users for a greener, cleaner future.
About the author: Robin van den Berg is Product Marketing Manager, Automotive at TomTom